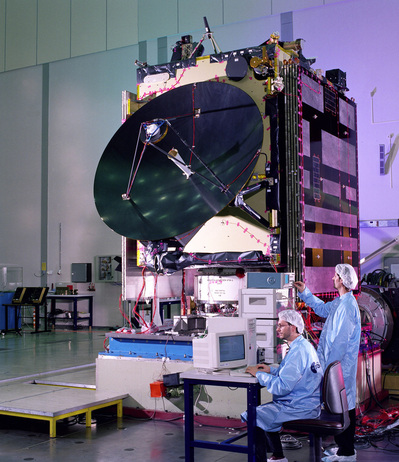
2014. Last Wednesday operators executed the first of 10 planned manoeuvres to accomplish orbit insertion about the comet nucleus on 6 August. This comprised an engine firing of about 45 minutes duration to refine Rosetta’s trajectory to ultimately bring the spacecraft and comet together. When I say ‘engine firing’, I am using the term loosely, as Rosetta doesn’t have a main rocket engine. Instead it has a collection of 24 thrusters, each with a thrust of 10 Newtons (10 N). Of these, 16 are
dedicated to attitude control – in other words they produce torques to rotate the spacecraft. Only the remaining 8 thrusters are used for actually changing the speed of the vehicle, which in turn changes its trajectory. A force of 10 N is about equivalent to the weight of a 1 kilogram bag of apples here on Earth, so the maximum level of thrust available for orbit manoeuvres is 80 N (or about 8 kg if you prefer). The low thrust gives an idea why the first post-hibernation burn took so long. The second planned burn will be larger, and take around 8 hours to complete.
So the next mission objective is to rendezvous with Comet C-G. The process of rendezvous requires not only that the spacecraft position and the comet’s position match, but also that their velocities are the same, therefore bringing them into a state of effectively zero relative velocity. During earlier parts of the mission (see blog Rosetta mission preview Part 2, March 31, 2014) the spacecraft encountered various planets and asteroids, but these were fly-bys where position was approximately matched but relative speed was significant. The process of
rendezvous is more complex, requires greater precision, and is also more expensive in terms of onboard resources (in particular propellant). This is why Rosetta has over a tonne and half of rocket propellant on board.

So what do I mean by a low-energy environment? Well the nucleus of Comet C-G is estimated (from remote observation) to be about 4 km in‘diameter’, so that its gravity field will be very weak. Consequently, a spacecraft in orbit around it will move at a very low speed. So for example, if Rosetta enters an initial orbit around Comet C-G with a radius of 100 km, then the circular orbit speed will be about 20 cm/sec (depending upon the density of the nucleus material). It’s likely that the initial orbit will be quite large, as the irregularities in the gravity
field get less the further away you are from the object. So this does give an idea of how sensitively the Rosetta spacecraft’s speed needs to be matched to that of the comet to achieve orbit. The escape velocity in this orbit will be around 30 cm/sec, so you can see that it would be easy for Rosetta to fail orbit insertion if there are even
small errors in speed. This is why Rosetta’s propulsion system has been design around the use of small thrusters – so that the speed precision that can be achieved is of the order of 1 mm/sec. The process of rendezvous
and orbit insertion is huge challenge for the spacecraft operators!
Having achieved such an orbit, the spacecraft would then be spend time observing the comet nucleus to quantify its irregular shape and to produce a ‘map’ of the gravity field. Once this has been done to a specified accuracy, the orbit can be reduced in radius to, say, 10 km from which the lander can be deployed. In this closer trajectory, the circular orbit speed increases to around 60 cm/sec, and the effects of the irregular gravity field become more pronounced. Close orbits around irregularly-shaped, rotating objects will not be confined to a single plane (as is the case for a spherical object) and may result in an impact with the surface after a number of orbital revolutions. This is why the period of observation and study in the higher initial orbit is required so that a lower orbit can be chosen that is stable. Once safely in the lower orbit, a further period of observation of the comet is required to refine knowledge of its gravity field, and to choose a scientifically promising site for Rosetta’s lander, which has been christened ‘Philae’. Since Philae will fall under gravity to the surface, a good knowledge of the comet’s gravity field is required in order to hit the desire landing site within a specified error.
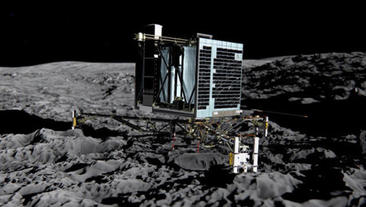
scientists will want to be ‘close to the action’ to maximise scientific return from Rosetta’s remote sensing instruments, particularly as the comet nears the Sun and plumes of gas and dust begin to erupt from the surface. On the other hand, the engineers will want a higher orbit to keep the
spacecraft safe and uncontaminated. Although I don’t know the precise mission plan, I would guess that a low
orbit radius of about 10 km (orbit altitude of about 5 km) would be likely.
If all goes well, the deployment of the Philae lander is planned in November 2014. If it freefalls from a height of, say, 5 km it will take about 2 to 3 hours to reach the surface, and will have an impact speed of around 1.5 m/sec at the surface (although details do depend upon the size and density of the nucleus – which are not known prior
to arrival). Another detail which is unknown until the moment of contact is the nature of the surface – it may be
‘bouncy’ or ‘sticky’,or something in between. The mass of the lander is about 100 kg, but in the low gravity at the surface of the comet it will weigh something like a 1/10th of a Newton, which is the weight of about 10 grams here on Earth. Given that the lander may bounce off the surface upon impact, some way of securing the lander to the surface is required to achieve a stable landing. The design includes the use of harpoons to secure the vehicle to the surface, and the legs are designed to dampen the initial impact. Communications with Earth to transmit science and image data will use the orbiting ‘mother spacecraft’ as a relay station to reduce the electrical power needed. The mission lifetime on the surface is planned to be at least one week, but an extended mission lasting months is may be possible.
Once all this is completed, the mission will continue through 13 August 2015 when the comet reaches perihelion (closest approach to the Sun) and will end nominally on 31 December 2015. Once the lander has expired – through technical failure or as a result of damage caused by surface eruptions – the main orbiting Rosetta spacecraft will continue to observe at close quarters what happens to a comet as it warm up due to solar heating. Obviously the effects of this has been seen remotely from Earth many times with the production of a comet’s dusty and gaseous tail, but this has never been observed in-situ before.
The bottom line is that the Rosetta mission should give a huge return in our scientific understanding of comets and their role in the origin and history of the Solar System. However, the technical challenges to orbit and land on comet C-G are very significant, and I wish ESA and the spacecraft operators every success in the coming months. I hope this series of blogs previewing the Rosetta mission has been useful and has wetted your
appetite for what is to come.